Research
Overview
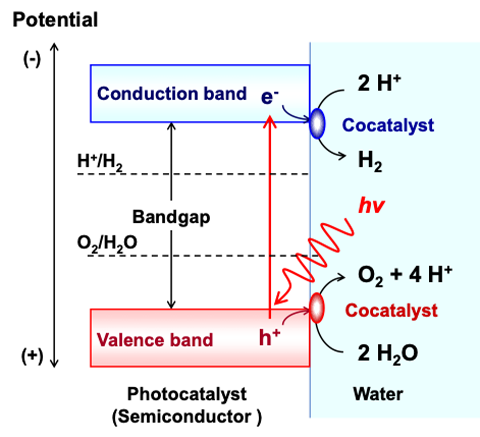
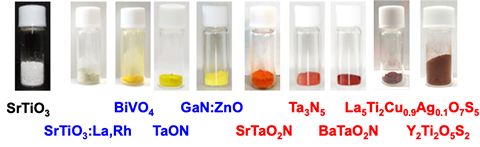
Modern societies rely on the mass consumption of energy resources, and most of the primary energy is derived from fossil resources. It is concerned that energy and environmental problems will become more serious on a global scale if our societies continue to develop while heavily relying on fossil resources. Hydrogen has been attracting attention as an energy alternative to fossil fuels. However, currently, fossil resources are used in various processes of hydrogen production. To solve energy and environmental problems from the root, it is necessary to develop technologies that can produce hydrogen using renewable energy without net consumption of fossil resources.
Sunlight-drive water splitting has been studied as a technology to produce renewable hydrogen. In our laboratory, we study particulate photocatalysts and reaction systems for sunlight-driven water splitting toward practical application of this technology. The photocatalysts considered here are semiconductors and absorb photons to generate negatively charged electrons and positively charged holes at excited states, which trigger redox reactions associated with water splitting. Systems based on particulate photocatalysts consists of relatively simple components and can be scaled up potentially by using inexpensive processes. Therefore, the development of photocatalysts highly active for the sunlight-drive water splitting reaction will be a major step towards practical large- scale hydrogen production.
To harvest solar energy effectively, it is necessary to develop photocatalysts that are active under visible light. In our laboratory, we mainly study particulate oxynitride, nitride, and oxysulfide semiconductors as photocatalyst materials. Many of these materials are known to strongly absorb long-wavelength visible light. To make these photocatalyst materials highly active, we investigate in detail correlations among synthesis and modification methods, physical properties, and photocatalysis of the materials. We also develop reaction systems suitable for large-scale applications taking advantage of the properties of particulate materials.
Reference(s)
https://www.nature.com/articles/s41929-019-0242-6
Oxide photocatalysts
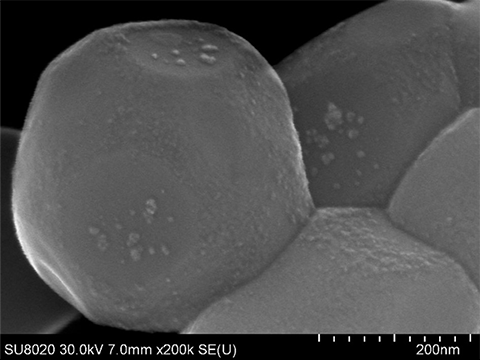
SrTiO3 is one of the particulate photocatalysts that was reported by Professor Kazunari Domen in 1980 to exhibit activity in the overall water splitting reaction for the first time in the world, and it is still well studied as a model water-splitting photocatalyst. Our laboratory found that the water-splitting activity of SrTiO3 was significantly improved by doping lower- valent metal cations. In particular, Al-doped SrTiO3 drove the overall water splitting reaction with external quantum efficiencies exceeding 90% in the near-ultraviolet region when site- selectively coloaded with hydrogen and oxygen evolution cocatalysts. This result demonstrates that even a particulate photocatalyst with a relatively simple structure can drive the endergonic water splitting reaction with a high quantum efficiency comparable to that of the light/chemical energy conversion process in natural photosynthesis and has a very important meaning in designing and developing particulate photocatalysts. The developed photocatalyst was used in a 100m2 scale water-splitting photocatalyst panel reaction system described later, pushing the research and development forward.
Reference(s)
https://pubs.rsc.org/en/content/articlelanding/1980/c3/c39800000543
https://www.nature.com/articles/s41586-020-2278-9
Oxynitride and nitride photocatalysts
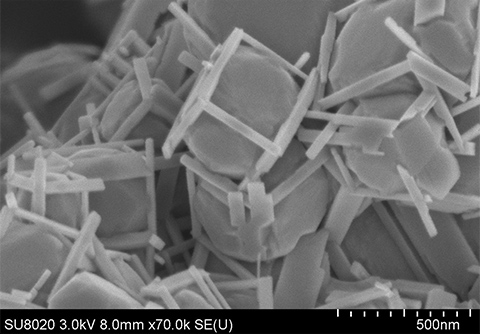
It has been known since around 2000 that oxynitrides and nitrides shows activity in hydrogen and oxygen evolution reactions from aqueous solutions under visible light irradiation. Ta3N5 is one of such nitride materials and absorbs visible light with wavelengths of up to 600nm. However, due to the difficulty in synthesizing high-quality Ta3N5 particles, overall water splitting had not been accomplished using Ta3N5 photocatalysts. In our laboratory, we surveyed the starting materials and synthesis conditions for Ta3N5 and produced Ta3N5 single-crystal nanorods that were active in the overall water splitting reaction. This Ta3N5 was obtained by nitriding KTaO3 for a short time. Ta3N5 single crystals with a low defect density were directly formed on the KTaO3 particles during the nitridation with gradual evaporation of the K component. Loading of an appropriate hydrogen evolution cocatalyst enabled the single-crystal Ta3N5 nanorods to utilize photoexcited charge carriers for the water splitting reaction.
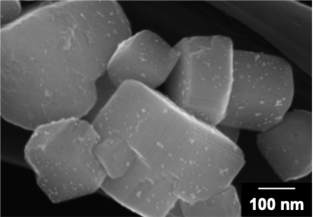
BaTaO2N is an oxynitride material that absorbs visible light up to 650nm. However, it was difficult to deposit cocatalysts with high dispersion and intimate contact, and photoexcited charge carriers were not utilized efficiently for the hydrogen evolution reaction. In our laboratory, we found that highly-dispersed Pt cocatalyst particles could be loaded on single-crystalline BaTaO2N particles synthesized by a flux method by applying an impregnation/hydrogen reduction method and a photodeposition method sequentially. The resultant Pt-loaded BaTaO2N photocatalyst exhibited significantly improved hydrogen evolution activity and also improved the efficiency of the two-step excitation(Z-scheme) water splitting reaction in combination with an oxygen generation photocatalyst. It was found by transient absorption spectroscopy that recombination of electrons and holes was suppressed because the Pt cocatalyst particles loaded by this method efficiently extracted electrons from the BaTaO2N photocatalyst. It was also found that the use of BaTaO2N with a low defect density was important for loading highly dispersed Pt cocatalysts.
Reference(s)
https://www.nature.com/articles/s41929-018-0134-1
https://www.nature.com/articles/s41467-021-21284-3
Oxysulfide photocatalysts
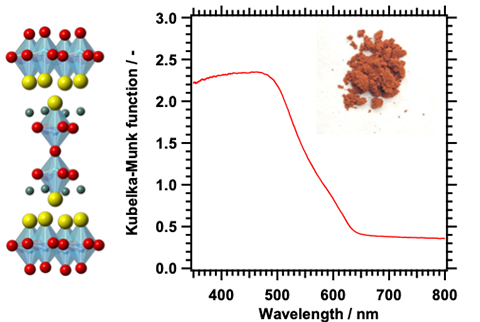
Oxysulfides are more stable than sulfides in water under light irradiation, and some materials have been known to have a band structure suitable for water splitting into hydrogen and oxygen in a weakly alkaline aqueous solution since around 2000. However, overall water splitting using oxysulfide photocatalysts had not been achieved for many years due to the lack of synthesis and cocatalyst loading suitable for photocatalytic applications. Through survey of stable oxysulfide photocatalyst materials, our laboratory succeeded in the over water splitting reaction using Y2Ti2O5S2, a layered perovskite-type oxysulfide absorbing visible light with wavelengths of up to 640 nm. To achieve the reaction, it was necessary to coload Y2Ti2O5S2 with Cr2O3-coated Rh as a hydrogen evolution cocatalyst and IrO2 as an oxygen evolution cocatalyst and to precisely control the pH value of the reaction solution. Further improvements in the performance are expected through refinements in the preparation methods of oxysulfide materials and cocatalysts.
Reference(s)
https://www.nature.com/articles/s41563-019-0399-z
Photocatalyst sheets
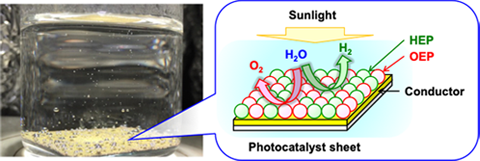
It is possible to split water into hydrogen and oxygen using two-step photoexcitation by combining hydrogen evolution photocatalysts (HEPs) and oxygen evolution photocatalysts (OEPs). Such photocatalytic systems are called Z-scheme systems after the photoexcitation process of natural photosynthesis. In our laboratory, we applied the particle transfer method, a method to fabricate particulate electrodes with low contact resistance, to prepare Z-schematic water-splitting particulate photocatalyst sheets in which HEPs and OEPs were blended and fixed on a thin film of conductive material. A photocatalyst sheet based on La,Rh co-doped SrTiO3 as a HEP and Mo-doped BiVO4 as an OEP immobilized on an Au thin film exhibited high water-splitting activity even in pure water. Notably, the photocatalyst sheet could maintain the water-splitting activity even when they were expanded to a large area, because evolution of hydrogen and oxygen occurred in close proximity, and the influence of solution resistance and mass transfer resistance could be minimized. In addition, we found that the excellent water-splitting performance was maintained even under atmospheric pressure, which was assumed to be practical conditions, when materials effective in preventing reverse reactions were applied. The solar-to-hydrogen energy conversion efficiency at the atmospheric pressure reached 1.0%, the highest ever reported using particulate photocatalysts. We will continue to develop photocatalyst sheets for water splitting using non-oxide photocatalyst materials, aiming for effective use of visible light with longer wavelengths.
Reference(s)
https://www.nature.com/articles/nmat4589
https://pubs.acs.org/doi/10.1021/jacs.6b12164
Photocatalyst panel reaction systems
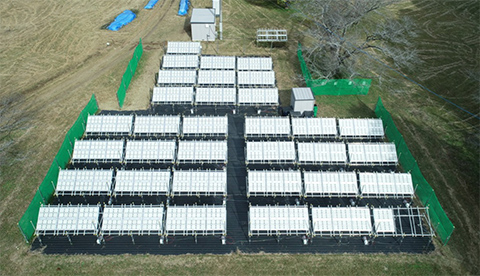
In collaboration with the Domen Laboratory of the University of Tokyo, our laboratory develops panel reaction systems toward large-scale application of particulate photocatalysts for water splitting. The aforementioned Al-doped SrTiO3 photocatalyst can be readily processed into sheet by coating onto substrates. It has been confirmed that such photocatalyst sheets housed in a panel reactor can decompose water continuously and transport the generated gas smoothly even when the water thickness is merely 0.1mm. In 2019, the construction of large-area water-splitting panel reaction systems was started, and the operating characteristics of the photocatalyst panels and associated equipment are under investigation for a long time. The reaction system, which consisted of a photocatalytic panel reactor with a light-receiving area of 100m2 and a gas separation module based on separation membranes, continuously decomposed water for about one year in an outdoor environment. In addition, by connecting the gas separation module, hydrogen gas was separated from the generated hydrogen-oxygen mixed gas with a purity of 94% at a recovery rate of more than 70%. Furthermore, by installing a mechanism controlling the gas flow rate in the gas separation module autonomously, the gas separation performance was maintained regardless of the sunshine conditions. Further research is in progress to reduce the cost and increase the scale of photocatalyst panel reactors and improve the separation performance and energy efficiency of the gas separation processes.
Reference(s)
https://www.nature.com/articles/s41586-021-03907-3